Хэрэглэгч:Bilguun.alt/Ноорог/Генетик
Загвар:Otheruses4 Загвар:Seeintro

Генетик бол амьд организмуудын хэлбэр болон удамшилыг судалдаг шинжлэх ухаан юм.[1][2] Балар эртний үеээс сонгон үржүүлэх аргаар ургамал болон амьтаныг сайжруулах зорилгоор онцлог шинж чанарын удамшилын талаарх мэдлэг далдуур хуримтлагдсаар ирсэн юм. Гэхдээ удамшилын механизмыг олж таних зорилготой орчин үеийн генетикийн шинжлэх ухаан нь 1800-аад оны дунд үед амьдарч байсан Грегор Менделийн хийсэн ажилаас улбаалдаг.[3] Хэдий тэрээр удамшилын зүй тогтолын физик суурь тулгуурыг мэддэгүй байсан ч Мендел удамшилын тулгуур нь тодорхой шинж чанар хоорондоо хамааралгүй замаар удамших дискрет процесс болохыг ажигласан байна. Удамшилын эдгээр тулгуур нэгжүүдийг өнөө үед гени гэж нэрлэдэг.
1900-аад оны эхэн үед Менделийн хийсэн ажиглалтуудыг дахин туршиж нээсний дараа 1910-аад оны үеээр эхэлсэн судалгааны үр дүнд анх удаа удамшилын тухай физик ойлголтыг гаргаж ирсэн. Том эслэг бүтэц болох хромосом гэгчийн дагуу шулуун байрласан гениүд байдаг тухай ойлголт юм. 1950-аад он гэхэд хромосомын цөм нь ДНХ гэж нэрлэгдэх болсон урт молекул байдаг болон гениүд нь энэхүү молекулын дотор шугаман хэсэг байдлаар оршдог болохыг ойлгосон. ДНХ-ийн нэг хэлхээ нь дөрвөн төрлийн нуклеотидоос бүрдсэн гинжин бүтэцтэй бөгөөд удамшилын мэдээлэл нь эдгээр нуклеотидуудын дараалалд агуулагддаг. 1953 онд Ватсон, Вилкинс, Крик нар ДНХ-ийн гурван хэмжээст бүтэц нь хос хэлхээ бүхий спираль бөгөөд хэлхээ тус бүр дээрх нуклеотидууд нь хос хосоороо холбогдон оршдогийг гаргасан байна. Хэлхээ бүр нь шинээр хам хэлхээ үүсгэх нийлэгжилтэнд зориулж загвар болж өгдөг бөгөөд мэдээлэлийг өвлүүлэх физик механизм нь болдог.
ДНХ дэхь нуклеотидын дараалал нь протейнуудыг үүсгэдэг амин хүчлийн тусгай дараалалыг бий болгоход ашиглагддаг. Үүнийг "генетик код" гэдэг. Протейн дахь энэ амин хүчлийн дараалал нь гурван хэмжээст бүтцэд хэрхэн эвхэгдэн хувирах ыг зохицуулдаг бөгөөд энэ бүтэц нь эргээд протейны ажиллагааг хариуцдаг. Протейн нь эсийн дотоод дахь бараг бүх л ажиллагааг хариуцдаг. ДНХ-ийн дараалалд гарсан өөрчлөлт нь протейны бүтэц болон ажиллагааг өөрчлөж чадах бөгөөд энэ нь улмаар эсийн хувьд болон тухайн бүхэл организмын хувьд томоохон өөрчлөлтийг бий болгож болно.
Генетик нь хэдий организмын зан төлөв болон шинж чанарыг тодорхойлоход гол үүрэгтэй оролцдог ч энэ нь мөн организмын хүрээлэн буй орчинтойгоо харилцсаны үр дүнд бий болох үр дагаварын харилцан үйлчлэлийг судалдаг. Жишээлбэл гени нь хүний өндөрийг тодорхойлоход гол үүрэгтэй байдаг бол мөн тэр хүний хүүхэд ахуй насанд учирсан эрүүл мэндийн нөхцөл, хир шим тэжээл авсан зэрэг нь үүнд ихээр нөлөөлдөг.
Генетикийн түүх
[засварлах | кодоор засварлах]
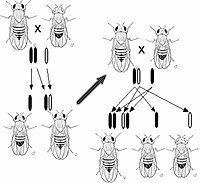
Генетикийн шинжлэх ухааны гарал үүсэл нь 19-р зууны дунд үед амьдарч байсан Грегор Менделийн ажилаас эхэлдэг ч удамшилын талаарх олон онол түүнээс өмнө хийгдэж байжээ. Эдгээр онолууд нь ерөнхийдөө олдмол шинж чанарын удамшил (мөн "сул удамшил" ч гэдэг) байдаг тухай авч үздэг. Энэ нь аливаа бодьгал нь түүний эцэг эхийн хүчтэй болсон, хөгжсөн шинж чанарыг өвлөн авдаг гэсэн итгэл үнэмшил байсан юм. Өнөө үед энэ онол нь ихэвчлэн Жеан-Баптист Ламарктай холбогддог. Тэрээр энэхүү удамшилын зүй тогтолыг ашиглан биологийн зүйлүүдийн доторх олон шинж чанарын хувьсалыг тайлбарласан юм (одоо үед эдгээр өөрчлөлтүүдийг сул удамшилын үр дүнд бий болдог гэхээс илүү байгалын шалгаралын үр дүн гэж үздэг).
Менделийн буюу сонгодог генетик
[засварлах | кодоор засварлах]Орчин үеийн генетикийн шинжлэх ухааны үндэс нь Герман Чех гаралтай Августины санваартан, эрдэмтэн Грегор Иоханн Менделийн хийсэн ажиглалтуудаас эхэлдэг юм. Тэрээр удамшилын зүй тогтолын талаар ургамал дээр нарийвчилсан судалгааг хийсэн билээ. Грегор Мендел 1865 онд Брунны байгалийн түүхийн нийгэмлэгт илтгэсэн өөрийн судалгааны ажил болох "Versuche über Pflanzenhybriden"-дээ ("Ургамалын эрлийзжүүлэлтийн туршилтууд") вандуйн ургамалын тодорхой шинж чанаруудын удамшилын зүй тогтолыг илрүүлж, математик аргаар илэрхийлж болохыг харуулсан байна.[4] Гэхдээ бүх онцлог шинжүүд Менделийн удамшилын эдгээр зүй тогтолыг дагадаггүй ч түүний ажил нь удамшилын судалгаанд статистикын хэрэглээг ашиглаж болох санааг гаргаж өгсөн юм.
Менделийг нас барсаны дараа түүнийхтэй ижил төрлийн судалгаа хийж байсан хэсэг эрдэмтэд түүний судалгааны ажилыг олж илрүүлэх хүртэл буюу 20-р зууны эхэн хүртэл түүний хийсэн ажиглалтын ач холбогдолыг хүн төрөлхтөн ойлгоогүй ирсэн юм. Менделийн судалгааны ажилыг маш ихээр дэмждэг, сурталчлагч Виллиам Бэйтсон "Генетик" гэдэг энэ үгийг анх 1905 онд Адам Сэджвикт бичсэн захидалдаа оруулж хэрэглэсэн юм.[5] "Генетик" гэдэг тэмдэг нэр нь (Грек хэлний γεννώ "генно" буюу төрүүлэх гэсэн утгатай үгнээс гаралтай) нэр үг болон хэрэглэгдэхээс өмнө 1830-аад оны үед анх хэрэглэгдэж эхэлсэн бөгөөд 1859 онд Чарлз Дарвин өөрийн Зүйлүүдийн гарал үүсэл номондоо анх удаа биологийн нэр томъёо болгон ашиглаж байжээ.[6] Бэйтсон 1906 онд Английн Лондон хотод болсон Ургамалын эрлийзжүүлэлтийн олон улсын гуравдугаар чуулган дээр өөрийн шинэ ажилын анхны илтгэлдээ удамшилын судалгааг "генетик" гэдэг үгээр тодорхойлж, энэхүү үгийн хэрэглээг түгээмэл болгосон.[7]
In the decades following rediscovery and popularization of Mendel's work, numerous experiments sought to elucidate the molecular basis of DNA. In 1910 Thomas Hunt Morgan argued that genes reside on chromosomes, based on observations of a sex-linked white eye mutation in fruit flies. In 1913 his student Alfred Sturtevant used the phenomenon of genetic linkage and the associated recombination rates to demonstrate and map the linear arrangement of genes upon the chromosome.
Молекул генетик
[засварлах | кодоор засварлах]Хромосом нь гениүдийг агуулдаг мөн хромосом нь протейн болон ДНХ хоёулангаас нь бүрэлддэг болохыг мэддэг байсан ч чухам аль нь удамшилын зүй тогтолд чухал нөлөөтөй эсвэл хэрхэн удамших процесс явагддаг болох нь тодорхойгүй байсан юм. 1928 онд Фредерик Гриффит өөрийн нээсэн хувирах үзэгдлийн тухай хэвлүүлсэн (Гриффитийн туршилтыг үзнэ үү.). Үүнээс 16 жилийн дараа 1944 онд Освальд Теодор Авери, Колин Маклеод, Мэклин Маккарти нар энэхүү үзэгдлийг ашиглан хувиралтанд чухал үүрэгтэй байдаг молекул буюу ДНХ-г тусгаарлаж, илрүүлж чадсан байна.[8] The Hershey-Chase experiment in 1952 identified DNA (rather than protein) as the genetic material of viruses, further evidence that DNA was the molecule responsible for inheritance.
James D. Watson and Francis Crick resolved the structure of DNA in 1953, using the X-ray crystallography work of Rosalind Franklin that indicated the molecule had a helical structure. Their double-helix model paired a sequence of nucleotides with a "complement" on the other strand. This structure not only provided a physical explanation for information contained within the order of the nucleotides, but also a physical mechanism for duplication through separation of strands and the reconstruction of a partner strand based on the nucleotide pairings. Although the structure explained the process of inheritance, it was still unknown how DNA influenced the behavior of cells. In the following years many scientists sought to understand how DNA controls the process of protein production within ribosomes, eventually discovering the transcription of DNA into messenger RNA and uncovering the genetic code which links the nucleotide sequence of messenger RNA to the amino acid sequence of protein.
With this molecular understanding of DNA, an explosion of research based on this understanding of the molecular nature of DNA became possible. The development of chain-termination DNA sequencing in 1977 enabled the determination of nucleotide sequences on DNA,[9] and the PCR method developed by Kary Banks Mullis in 1983 allowed the isolation and amplification of arbitrary segments of DNA.[10] These and other techniques, through the pooled efforts of the Human Genome Project and parallel private effort by Celera Genomics, culminated in the sequencing of the human genome in 2001.
Удамшилын онцлогууд
[засварлах | кодоор засварлах]Дискрет (бүхэл) удамшил ба Менделийн хуулиуд
[засварлах | кодоор засварлах]

Хамгийн тулгуур түвшинд организмын удамшил нь "гени" хэмээгдэх дискрет шинж чанаруудаар илэрдэг.[11] Энэхүү шинж чанарыг анх Грегор Мендел вандуйн ургамалын удамших шинж чанаруудыг тусгаарлаж судалж байхдаа ажиглажээ.[4][12] Цэцгийн өнгөний шинж чанарыг судалж байсан туршилтаараа Мендел вандуйн ургамал бүрийн цэцгүүд нэг бол ягаан эсвэл цагаан өнгөтэй бөгөөд хоёр өнгөний дундын хувилбар ерөөсөө олдохгүйг ажигласан байна. Ижилхэн генийн эдгээр өөр, дискрет хувилбаруудыг "аллеле" гэдэг.
Вандуйн ургамалын хувьд организм бүрийн гени болгонд хоёр аллеле байдаг бөгөөд ургамал нь эх эцэг ургамал бүрээс нэг нэг аллелийг өвлөн авдаг байна.[13] Хүмүүсээс эхлэн олон организмуудад энэхүү удамшилын зүй тогтол байдаг. Ижил аллелийн хоёр хуулбартай организмуудыг "хомозигос" гэдэг бол хоёр өөр аллеле бүхий организмуудыг "гетерозигос" гэдэг.
Тухайн организмын аллелийн цогцыг түүний генотип гэдэг бол харин организмд харагдах шинж чанар байвал түүнийг нь тухайн организмын "финотип" гэдэг. Организм нь гетерозигос байх тохиолдолд түүний нэг аллелийг ихэвчлэн "давамгайлагч" гэдэг бөгөөд учир нь тэрхүү аллелийн шинж чанар нь тухайн организмын финотип дээр "давамгайлдаг". Харин нөгөө аллелийг нь "рецессив" гэх бөгөөд түүний шинж чанар нь "бүдэг" бөгөөд ажиглагддаггүй. Давамгайлагч аллелуудыг гол төлөв том үсгээр товчилон тэмдэглэдэг бол рецессив аллелийг тэрхүү үсгийн жижиг хувилбараар нь тэмдэглэдэг.[14] Зарим аллелийн хувьд бүрэн гүйцэд давамгайлал байдаггүй бөгөөд үүний оронд дундын финотипыг илэрхийлэх замаар бүрэн бус давамгайлалтай байдаг эсвэл хоёр аллелийг нэгэн зэрэг илэрхийлэх замаар давхар давамгайлалтай байдаг.[15]
Эцэг эх хүүхдүүдтэй болоход тэдний хүүхдүүд нь санамсаргүйгээр эцэг эх тус бүрийн хоёр аллелаас нэг нэгийг нь өвлөн авдаг. Энэхүү сөөлжилтийг Пунетын квадратаар дүрслэн харуулж болно. Дискрет удамшил болон аллелуудын ялгаварлалтын үзэгдэлийг ерөнхийд нь "Менделийн нэгдүгээр хууль" гэдэг.
Assortment and interactions of multiple genes
[засварлах | кодоор засварлах]Organisms have thousands of genes, and in sexually reproducing organisms assortment of these genes are generally independent of each other. This means that the inheritance of an allele for yellow or green pea color is unrelated to the inheritance of alleles for white or purple flowers. This phenomenon, known as "Mendel's second law" or the "Law of independent assortment", means that the alleles of different genes get shuffled between parents to form children with many different combinations. (Some genes do not assort independently, demonstrating genetic linkage, a topic discussed later in this article.)
Often different genes can interact in a way that influences the same trait. In the blue-eyed Mary, for example, there exists a gene with alleles that determine the color of flowers: blue or magenta. Another gene, however, controls whether the flowers have color at all: color or white. When a plant has two copies of this white allele, its flowers are white — regardless of whether the first gene has blue or magenta alleles. This interaction between genes is called "epistasis", with the second gene epistatic to the first.[16]
Many traits are not discrete features (eg. purple or white flowers) but are instead continuous features (eg. human height and skin color). These "complex traits" are the product of interactions of many genes.[17] The influence of these genes is mediated, to varying degrees, by the environment an organism has experienced. The degree to which an organism's genes contribute to a complex trait is called "heritability".[18] Measurement of the heritability of a trait is relative, though — in a more variable environment, the environment has a bigger influence on the total variation of the trait. For example, human height is a complex trait with a heritability of 89% in the United States. In Nigeria, however, where people experience a more variable access to good nutrition and health care, height has a heritability of only 62%.[19]
Удамшилын молекул түвшин
[засварлах | кодоор засварлах]ДНХ болон хромосом
[засварлах | кодоор засварлах]
Гениүдийн молекул суурь нь Дезоксирибонуклейны хүчил (ДНХ) юм. ДНХ нь нуклеотидуудын гинжин хэлхээнээс тогтдог ба аденин (А), цитозин (Ц), гуанин (Г), тимин (Т) гэсэн дөрвөн янзын нуклеотид байдаг. Генетикийн мэдээлэл нь эдгээр нуклеотидын дараалалд орших бөгөөд гени нь ДНХ-ийн гинжний дагуух дараалалын хэсгүүд болон оршдог.[20] Зөвхөн вирусүүд л дээрхээс ялгаатай байдаг ба зарим тохиолдолд вирүсүүд ДНХ-ийн оронд түүнтэй тун төстэй молекул болох РНХ-г өөрсдийн генетикийн материал болгон ашигладаг.[21] Организм дахь бүх удамшилын материалын бүрэн цогцыг түүний "геном" гэдэг.
ДНХ нь гол төлөв хоёр-хэлхээт молекул байдлаар оршдог бөгөөд хос-спираль хэлбэрээр мушгирсэн байдаг. DNA normally exists as a double-stranded molecule, coiled into the shape of a double-helix. Each nucleotide in DNA preferentially pairs with its partner nucleotide on the opposite strand: A pairs with T, and C pairs with G. Thus, in its two-stranded form, each strand effectively contains all necessary information, redundant with its partner strand. This structure of DNA is the physical basis for inheritance: DNA replication duplicates the genetic information by splitting the strands and using each strand as a template for synthesis of a new partner strand.[22]
Genes are arranged linearly along the long chains of DNA sequence, called chromosomes. In bacteria, each cell has a single circular chromosome, while eukaryotic organisms (which includes plants and animals) have their DNA arranged in multiple linear chromosomes. These DNA strands are often extremely long; the largest human chromosome, for example, is about 247 million base pairs in length.[23] The DNA of a chromosome is associated with structural proteins which organize, compact, and control access to the DNA, forming a material called chromatin; in eukaryotes chromatin is usually composed of nucleosomes, repeating units of DNA wound around a core of histone proteins.[24] The full set of hereditary material in an organism (usually the combined DNA sequences of all chromosomes) is called the "genome".
While haploid organisms have only one copy of each chromosome, most animals and many plants are diploid, containing two copies of each chromosome and thus two copies of every gene.[25] The two alleles for a gene are located on identical loci of sister chromatids, each allele inherited from a different parent.

An exception exists in the sex chromosomes, specialized chromosomes many animals have evolved that play a role in determining the sex of the organism.[26] In humans and other mammals the Y chromosome has very few genes and triggers the development of male sexual characteristics, while the X chromosome is similar to the other chromosomes and contains many genes unrelated to sex determination. Females have two copies of the X chromosome, but males have one Y and only one X chromosome — this difference in X chromosome copy numbers leads to the unusual inheritance patterns of sex linked disorders.
Reproduction
[засварлах | кодоор засварлах]
When cells divide, their full genome is copied and each daughter cell inherits one copy. This is a simplest form of reproduction and is the basis for asexual reproduction. Asexual reproduction can also occur in multicellular organisms, always producing children which each inherit a full copy of a single parental genome. When asexual reproduction occurs, the child organisms are "clones" as they contain the same genetic material as the parent.
Eukaryotic organisms often use sexual reproduction to generate children which contain a mixture of genetic material inherited from two different parents. The process of sexual reproduction generally alternates between forms which contain single copies of the genome (haploid) and double copies (diploid).[25] Haploid cells fuse and combine genetic material to create a diploid cell with paired chromosomes. Diploid organisms form haploids by dividing, without replicating their DNA, to create daughter cells that randomly inherit one of each pair of chromosomes. Most animals and many plants are diploid for the majority of their lifespan, with the haploid form reduced to single cell gametes.

Although they do not use the haploid/diploid method of sexual reproduction, bacteria have many methods of acquiring new genetic information. Some bacteria can undergo conjugation, transferring a small circular piece of DNA to another bacterium.[27] Bacteria can also take up raw DNA fragments found in the environment and integrate them into their genome, a phenomenon known as transformation.[28] This processes result in horizontal gene transfer, transmitting fragments of genetic information between organisms that would otherwise be unrelated.
Genetic recombination and linkage
[засварлах | кодоор засварлах]
The diploid nature of chromosomes allows for genes on different chromosomes to assort independently during sexual reproduction, recombining to form new combinations of genes. Genes on the same chromosome would theoretically never recombine, however, were it not for the process of chromosomal crossover. During crossover, chromosomes exchange stretches of DNA, effectively shuffling the gene alleles between the chromosomes to .[29] This process of chromosomal crossover generally occurs during meiosis, a series of cell divisions that creates haploid germ cells which later combine with other germ cells to form child organisms.
The probability of chromosomal crossover occurring between two given points on the chromosome is related to the distance between them. For an arbitrarily long distance, the probability of crossover is high enough that the inheritance of the genes is effectively uncorrelated. For genes that are closer together, however, the lower probability of crossover means that the genes demonstrate genetic linkage — alleles for the two genes tend to be inherited together. The amounts of linkage between a series of genes can be combined to form a linear linkage map that roughly describes the arrangement of the genes along the chromosome.[30]
Gene expression and the creation of phenotype
[засварлах | кодоор засварлах]The genetic code
[засварлах | кодоор засварлах]
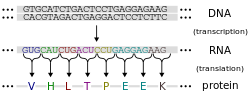
Genes generally express their functional effect through the production of proteins, which are complex molecules responsible for most functions in the cell.[31] Proteins are chains of amino acids, and the DNA sequence of a gene (through an RNA intermediate) is used to produce a specific protein sequence. Each group of three nucleotides in the sequence, called a codon, corresponds to one of the twenty possible amino acids in protein — this correspondence is called the genetic code.[32] The flow of information is unidirectional: information is transferred from nucleotide sequences into the amino acid sequence of proteins, but never from protein back into the sequence of DNA — a phenomenon Francis Crick called the central dogma of molecular biology.[33]
The specific sequence of amino acids results in a unique three-dimensional structure for that protein, and the three-dimensional structures of protein are related to their function.[34][35] Some are simple structural molecules, like the fibers formed by the protein collagen. Proteins can bind to other proteins and simple molecules, sometimes acting as enzymes by facilitating chemical reactions within the bound molecules (without changing the structure of the protein itself). Protein structure is dynamic; the protein hemoglobin bends into slightly different forms as it facilitates the capture, transport, and release of oxygen molecules within mammalian blood.
A single nucleotide difference within DNA can cause a single change in the amino acid sequence of a protein. Because protein structures are the result of their amino acid sequences, some changes can dramatically change the properties of a protein by destabilizing the structure or changing the surface of the protein in a way that changes its interaction with other proteins and molecules. For example, sickle-cell anemia is a human genetic disease that results from a single base difference within the coding region for the β-globin section of hemoglobin, causing a single amino acid change that changes hemoglobin's physical properties.[36] Sickle-cell versions of hemoglobin stick to themselves, stacking to form fibers that distort the shape of red blood cells carrying the protein. These sickle-shaped cells no longer flow smoothly through blood vessels, having a tendency to clot or degrade, causing the medical problems associated with the disease.
Nature vs. nurture
[засварлах | кодоор засварлах]Although genes contain all the information an organism uses to function, the environment plays an important role in determining the ultimate phenotype — a dichotomy often referred to as "nature vs. nurture". The phenotype of an organism depends on the interaction of genetics with the environment. One example of this is the case of temperature-sensitive mutations. Often, a single amino acid change within the sequence of a protein does not change its behavior and interactions with other molecules, but it does destabilize the structure. In a high temperature environment, where molecules are moving more quickly and hitting each other, this results in the protein losing its structure and failing to function. In a low temperature environment, however, the protein's structure is stable and functions normally. This sort of mutation is visible in the coat coloration of Siamese cats, where a mutation in an enzyme responsible for pigment production causes it to destabilize and lose function at high temperatures.[37] The protein remains functional in areas of skin that are colder — legs, ears, tail, and face — and so the cat has dark fur at its extremities.
Environment also plays a dramatic role in effects of the human genetic disease phenylketonuria.[38] The mutation that causes phenylketonuria disrupts the ability of the body to break down the amino acid phenylalanine, causing toxic build-up of an intermediate molecule that, in turn, causes severe symptoms of progressive mental retardation and seizures. If someone with the phenylketonuria mutation is kept on a strict diet that avoids this amino acid, however, they remain normal and healthy.
Gene regulation
[засварлах | кодоор засварлах]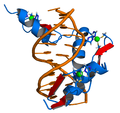
The genome of a given organism contains thousands of genes, but not all these genes need to be active at any given moment. A gene is "expressed" when it is being transcribed into mRNA (and translated into protein), and there exist many cellular methods of controlling the expression of genes such that proteins are produced only when needed by the cell. Transcription factors are regulatory proteins that bind to the start of genes, either promoting or inhibiting the transcription of the gene.[39] Within the genome of Escherichia coli bacteria, for example, there exists a series of genes necessary for the synthesis of the amino acid tryptophan. However, when tryptophan is already available to the cell, these genes for tryptophan synthesis are no longer needed. The presence of tryptophan directly affects the activity of the genes — the tryptophan molecules bind to the tryptophan repressor (a transcription factor), changing the repressor's structure such that the repressor is "active" and binding to the genes. The tryptophan repressor blocks the transcription and prevents expression of the genes, thus creating negative feedback regulation of the tryptophan synthesis process.[40]
Differences in gene expression are especially clear within multicellular organisms, where cells all contain the same genome but have very different structures and behaviors due to the expression of different sets of genes. All the cells in a multicellular organism derive from a single cell, differentiating into different cell types in response to external and intercellular signals and gradually establishing different patterns of gene expression to create different behaviors. No single gene is responsible for the development of structures within multicellular organisms, these patterns arise from the complex interactions between many cells.
Within eukaryotes there exist structural features of chromatin which influence the transcription of genes, often in the form of modifications to DNA and chromatin that are stably inherited by daughter cells.[41] These features are called "epigenetic" because they exist "on top" of the DNA sequence and retain inheritance from one cell generation to the next. Because of epigenetic features, different cell types grown within the same medium can retain very different properties. Although epigenetic features are generally dynamic over the development of organisms, some, like the phenomenon of paramutation, have multigenerational inheritance and exist as rare exceptions to the general rule of DNA as the basis for inheritance.[42]
Mutations and natural selection
[засварлах | кодоор засварлах]Mutations
[засварлах | кодоор засварлах]
During the process of DNA replication, errors occasionally occur in the polymerization of the second strand (these error rates are generally extremely low, 1 error in every 10-100 million bases).[43][44] These errors, called mutations, can have an impact on the phenotype of an organism, especially if they occur within the protein coding sequence of a gene. Processes which increase the rate of changes in DNA are called "mutagenic": mutagenic chemicals promote errors in DNA replication, often by interfering with the structure of base-pairing, while UV radiation induces mutations by causing damage to the DNA structure.[45] Chemical damage to DNA occurs naturally as well, and cells use DNA repair mechanisms to repair mismatches and breaks in DNA -- nevertheless, the repair sometimes fails to return the DNA to its original sequence.
In organisms which use chromosomal crossover to exchange DNA and shuffle genes, errors in alignment during meiosis can also cause mutations.[46] Errors in crossover are especially likely when similar sequences cause partner chromosomes to adopt a mistaken alignment, which makes some regions in genomes more prone to mutating in this way. These errors create large structural changes in DNA sequence -- duplications, inversions or deletions of entire regions, or the accidental exchanging of whole parts between different chromosomes (called "translocation").
Natural selection and evolution
[засварлах | кодоор засварлах]
Mutations produce organisms with different genotypes, and those differences can result in different phenotypes. Many genetic mutations, called "neutral mutations", have a negligible effect on an organism's phenotype, health, and reproductive fitness. Mutations which do have an effect are often deleterious, but occasionally mutations arise which are beneficial in the current environmental context of the organism.

Population genetics research studies the distributions of these genetic differences within populations and how the distributions change over time. Changes in the frequency of an allele in a population can be influenced by natural selection, where a given allele's higher rate of survival and reproduction causes it to become more frequent in the population over time. Genetic drift can also occur, where chance events lead to random changes in allele frequency.
Over many generations, the genomes of organisms can change, resulting in the phenomenon of evolution. Mutations and the selection for beneficial mutations can cause a species to evolve into forms that better survive their environment, a process called adaptation. New species are formed through the process of speciation, a process often caused by geographical separations that allow different populations to genetically diverge.
As sequences diverge and change during the process of evolution, these differences between sequences can be used as a molecular clock to calculate the evolutionary distance between them. Genetic comparisons are generally considered the most accurate method of characterizing the relatedness between species, an improvement over the sometimes deceptive comparison of phenotypic characteristics. The evolutionary distances between species can be combined to form evolutionary trees. These trees are commonly considered the most accurate representation of relatedness, although the transfer of genetic material between unrelated species (known as "horizontal gene transfer" and most common in bacteria) cannot be represented by the tree.
Genetic research and technology
[засварлах | кодоор засварлах]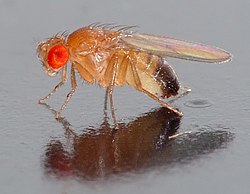
Model organisms and genetics
[засварлах | кодоор засварлах]Although geneticists originally studied inheritance in a wide range of organisms, researchers began to specialize in studying the genetics of a particular subset of organisms. The fact that significant research already existed for a given organism would encourage new researchers to choose it for further study, and so eventually a few "model organisms" became the basis for most genetics research.[47] Common research topics in model organism genetics include the study of gene regulation and the involvement of genes in development and cancer.
Organisms were chosen, in part, for convenience — short generation times and facile genetic manipulation made some organisms popular genetics research tools. Widely used model organisms include the gut bacterium Escherichia coli, the plant Arabidopsis thaliana, baker's yeast (Saccharomyces cerevisiae), the nematode Caenorhabditis elegans, the common fruit fly (Drosophila melanogaster), and the common house mouse (Mus musculus).
Medical genetics research
[засварлах | кодоор засварлах]Medical genetics research seeks to find and study the genetic causes of human diseases.[48] When searching for an unknown gene that may be involved in a disease, researchers commonly use genetics linkage and genetic pedigree charts to find the location on the genome associated with the disease. At the population level, researchers take advantage of Mendelian randomization to look for locations in the genome that are associated with diseases, a technique especially useful for multigenic traits not clearly defined by a single gene. Once a candidate gene is found, further research is often done on the same gene (called an orthologous gene) in model organisms.
Although many inherited diseases are subjects of genetic research, cancer is also considered a genetic disease.[49] The process of cancer development in the body is a combination of events. Mutations occasionally occur within cells in the body as they divide — while these mutations will not be inherited by any children, they can effect the behavior of cells, sometimes causing them to grow and divide more frequently. There are biological mechanisms that attempt to stop this process — signals are given to inappropriately dividing cells that should trigger cell death, but sometimes additional mutations occur that cause cells to ignore these messages. An internal process of natural selection occurs within the body and eventually mutations accumulate within cells to promote their own growth, creating a cancerous tumor that grows and invades various tissues of the body.
Genetic technologies
[засварлах | кодоор засварлах]
A variety of techniques exist for manipulating DNA in the laboratory. Restriction enzymes are a commonly used enzyme that cuts DNA at specific sequences, producing predictable fragments of DNA.[50] The use of ligation enzymes allows these fragments to be stitched back together, and by ligating fragments of DNA together from different sources, researchers can create recombinant DNA. Often associated with genetically modified organisms, recombinant DNA is commonly used in the context of plasmids — short circular DNA fragments with a few genes on them. By inserting plasmids into bacteria and growing those bacteria on plates of agar (to isolate clones of bacteria cells), researchers can clonally amplify the inserted fragment of DNA (a process known as molecular cloning). (Cloning can also refer to the creation of clonal organisms, through various techniques.)
DNA can also be amplified using a procedure called the polymerase chain reaction (PCR).[51] By using specific short sequences of DNA, PCR can exponentially amplify a targeted region of DNA, isolating and amplifying a selected section of DNA. Because it can amplify from extremely small amounts of DNA, PCR is also often used to detect the presence of specific DNA sequences.
DNA sequencing & genomics
[засварлах | кодоор засварлах]One of the most fundamental technologies developed to study genetics, DNA sequencing allows researchers to determine the sequence of nucleotides in DNA fragments. Developed in 1977 by Frederick Sanger and coworkers, chain-termination sequencing is now routinely used to sequence DNA fragments.[52] With this technology, researchers have been able to study the molecular sequences associated with many human diseases. As sequencing has become less expensive and with the aid of computational tools, researchers have sequenced the genomes of many organisms by stitching together the sequences of many different fragments (a process called "genome assembly").[53] These technologies were used to sequence the human genome, leading to the completion of the Human genome project in 2003.[54]
The large amount of sequences available has created the field of "genomics," research which uses computational tools to search for and analyze patterns in the full genomes of organisms. Genomics can also be considered a subfield of bioinformatics, which uses computational approaches to analyze large sets of biological data.
References
[засварлах | кодоор засварлах]- ↑
Griffiths AJF, Miller JH, Suzuki DT, Lewontin RC, and Gelbart WM (2000). An Introduction to Genetic Analysis. New York: W.H. Freeman and Company.
{{cite book}}
: CS1 maint: multiple names: authors list (link) ISBN 0-7167-3520-2. - ↑ Hartl D & Jones E (2005). Genetics: Analysis of Genes and Genomes, 6th edition. Jones & Bartlett. 854 pages. ISBN 0-7637-1511-5.
- ↑ Weiling F (1991). "Historical study: Johann Gregor Mendel 1822-1884". American Journal of Medical Genetics. 40 (1): 1–25, discussion 26. PMID 1887835.
- ↑ 4.0 4.1 Mendel, GJ (1866). "Versuche über Pflanzen-Hybriden". Verhandlungen des naturforschenden Vereins Brünn. 4: 3–47. (in English in 1901, J. R. Hortic. Soc. 26: 1–32) translation available online
- ↑ Online copy of William Bateson's letter to Adam Sedgwick
- ↑ genetic, a. and n. pl., Oxford English Dictionary, 2nd ed. (1989)
- ↑ Bateson, W (1907). "The Progress of Genetic Research". Wilks, W (editor) Report of the Third 1906 International Conference on Genetics: Hybridization (the cross-breeding of genera or species), the cross-breeding of varieties, and general plant breeding, London: Royal Horticultural Society.
- Although the conference was titled "International Conference on Hybridisation and Plant Breeding", Wilks changed the title for publication as a result of Bateson's speech.
- ↑ Avery OT, MacLeod CM, and McCarty M (1944). "Studies on the Chemical Nature of the Substance Inducing Transformation of Pneumococcal Types: Induction of Transformation by a Desoxyribonucleic Acid Fraction Isolated from Pneumococcus Type III". Journal of Experimental Medicine. 79 (1): 137–58.
{{cite journal}}
: CS1 maint: multiple names: authors list (link)35th anniversary reprint available - ↑
Sanger F, Nicklen S, and Coulson AR (1977). "DNA sequencing with chain-terminating inhibitors". Nature. 74 (12): 5463–5467.
{{cite journal}}
: CS1 maint: multiple names: authors list (link) - ↑
Saiki RK, Scharf S, Faloona F, Mullis KB, Horn GT, Erlich HA, Arnheim N (1985). "Enzymatic Amplification of β-Globin Genomic Sequences and Restriction Site Analysis for Diagnosis of Sickle Cell Anemia". Science. 230 (4732): 1350–1354.
{{cite journal}}
: CS1 maint: multiple names: authors list (link) - ↑
Griffiths AJF, Miller JH, Suzuki DT, Lewontin RC, and Gelbart WM (2000). An Introduction to Genetic Analysis. W.H. Freeman and Company.
{{cite book}}
: CS1 maint: multiple names: authors list (link) Chapter 2 (Patterns of Inheritance): Introduction - ↑
Griffiths AJF, Miller JH, Suzuki DT, Lewontin RC, and Gelbart WM (2000). An Introduction to Genetic Analysis. W.H. Freeman and Company.
{{cite book}}
: CS1 maint: multiple names: authors list (link) Chapter 2 (Patterns of Inheritance): Mendel's experiments - ↑
Griffiths AJF, Miller JH, Suzuki DT, Lewontin RC, and Gelbart WM (2000). An Introduction to Genetic Analysis. W.H. Freeman and Company.
{{cite book}}
: CS1 maint: multiple names: authors list (link)Chapter 3 (Chromosomal Basis of Heredity): Mendelian genetics in eukaryotic life cycles - ↑ This form of notation is especially common in plants. There are other types of notation, you can read more here.
- ↑
Griffiths AJF, Miller JH, Suzuki DT, Lewontin RC, and Gelbart WM (2000). An Introduction to Genetic Analysis. W.H. Freeman and Company.
{{cite book}}
: CS1 maint: multiple names: authors list (link) Chapter 4 (Gene Interaction): Interactions between the alleles of one gene - ↑
Griffiths AJF, Miller JH, Suzuki DT, Lewontin RC, and Gelbart WM (2000). An Introduction to Genetic Analysis. W.H. Freeman and Company.
{{cite book}}
: CS1 maint: multiple names: authors list (link) Chapter 4 (Gene Interaction): Gene interaction and modified dihybrid ratios - ↑ Mayeux R (2005). "Mapping the new frontier: complex genetic disorders". The Journal of Clinical Investigation. 115 (6): 1404–7. PMID 15931374.
- ↑ Griffiths AJF, Miller JH, Suzuki DT, Lewontin RC, and Gelbart WM (2000). An Introduction to Genetic Analysis. W.H. Freeman and Company.
{{cite book}}
: CS1 maint: multiple names: authors list (link) Chapter 25 (Quantitative Genetics): Quantifying heritability - ↑ Luke A, Guo X, Adeyemo AA, Wilks R, Forrester T, Lowe W Jr, Comuzzie AG, Martin LJ, Zhu X, Rotimi CN, Cooper RS (2001). "Heritability of obesity-related traits among Nigerians, Jamaicans and US black people". Int J Obes Relat Metab Disord. 25 (7): 1034–1041.
{{cite journal}}
: CS1 maint: multiple names: authors list (link) Abstract from NCBI - ↑ Pearson H (2006). "Genetics: what is a gene?". Nature. 441 (7092): 398–401. PMID 16724031.
- ↑ Prescott, L (1993). Microbiology. Wm. C. Brown Publishers. 0-697-01372-3.
- ↑ A.J.F. Griffiths, J.H. Miller, D.T. Suzuki, R.C. Lewontin, and W.M. Gelbart (2000). An Introduction to Genetic Analysis. W.H. Freeman and Company.
{{cite book}}
: CS1 maint: multiple names: authors list (link)Chapter 8 (The Structure and Replication of DNA): Mechanism of DNA Replication - ↑ S. G. Gregory; et al. (2006). "The DNA sequence and biological annotation of human chromosome 1". Nature. 441.
{{cite journal}}
: Explicit use of et al. in:|author=
(help); Unknown parameter|page-=
ignored (help) free full text available - ↑
Alberts B, Johnson A, Lewis J, Raff M, Roberts K, and Walter P (2002). Molecular Biology of the Cell (4th edition ed.).
{{cite book}}
:|edition=
has extra text (help)CS1 maint: multiple names: authors list (link) DNA and chromosomes: Chromosomal DNA and Its Packaging in the Chromatin Fiber - ↑ 25.0 25.1 A.J.F. Griffiths, J.H. Miller, D.T. Suzuki, R.C. Lewontin, and W.M. Gelbart (2000). An Introduction to Genetic Analysis. W.H. Freeman and Company.
{{cite book}}
: CS1 maint: multiple names: authors list (link)Chapter 3 (Chromosomal Basis of Heredity): Mendelian genetics in eukaryotic life cycles - ↑ A.J.F. Griffiths, J.H. Miller, D.T. Suzuki, R.C. Lewontin, and W.M. Gelbart (2000). An Introduction to Genetic Analysis. W.H. Freeman and Company.
{{cite book}}
: CS1 maint: multiple names: authors list (link)Chapter 2 (Patterns of Inheritance): Sex chromosomes and sex-linked inheritance - ↑ A.J.F. Griffiths, J.H. Miller, D.T. Suzuki, R.C. Lewontin, and W.M. Gelbart (2000). An Introduction to Genetic Analysis. W.H. Freeman and Company.
{{cite book}}
: CS1 maint: multiple names: authors list (link)Chapter 7 (Gene Transfer in Bacteria and Their Viruses): Bacterial conjugation - ↑ A.J.F. Griffiths, J.H. Miller, D.T. Suzuki, R.C. Lewontin, and W.M. Gelbart (2000). An Introduction to Genetic Analysis. W.H. Freeman and Company.
{{cite book}}
: CS1 maint: multiple names: authors list (link)Chapter 7 (Gene Transfer in Bacteria and Their Viruses): Bacterial transformation - ↑ A.J.F. Griffiths, J.H. Miller, D.T. Suzuki, R.C. Lewontin, and W.M. Gelbart (2000). An Introduction to Genetic Analysis. W.H. Freeman and Company.
{{cite book}}
: CS1 maint: multiple names: authors list (link)Chapter 5 (Basic Eukaryotic Chromosome Mapping): Nature of crossing-over - ↑ A.J.F. Griffiths, J.H. Miller, D.T. Suzuki, R.C. Lewontin, and W.M. Gelbart (2000). An Introduction to Genetic Analysis. W.H. Freeman and Company.
{{cite book}}
: CS1 maint: multiple names: authors list (link)Chapter 5 (Basic Eukaryotic Chromosome Mapping): Linkage maps - ↑ Some genes are transcribed into RNA, but their RNA products are never used to produce protein. These RNA products may fold into forms with enzymatic properties (eg. ribosomal RNA and transfer RNA), or they may have a regulatory effect through hybridization interactions with other RNA molecules (eg. microRNA).
- ↑ J.M. Berg, J.L. Tymoczko, L. Stryer, N.D. Clarke (2002). Biochemistry (5th edition ed.). New York: W. H. Freeman and Company.
{{cite book}}
:|edition=
has extra text (help)CS1 maint: multiple names: authors list (link) I. 5. DNA, RNA, and the Flow of Genetic Information: Amino Acids Are Encoded by Groups of Three Bases Starting from a Fixed Point - ↑ Crick, F. (1970): Central Dogma of Molecular Biology. Nature 227, 561-563. PMID 4913914
- ↑
Alberts B, Johnson A, Lewis J, Raff M, Roberts K, and Walter P (2002). Molecular Biology of the Cell (4th edition ed.).
{{cite book}}
:|edition=
has extra text (help)CS1 maint: multiple names: authors list (link) Proteins: The Shape and Structure of Proteins - ↑
Alberts B, Johnson A, Lewis J, Raff M, Roberts K, and Walter P (2002). Molecular Biology of the Cell (4th edition ed.).
{{cite book}}
:|edition=
has extra text (help)CS1 maint: multiple names: authors list (link) Proteins: Protein Function - ↑ Kenneth R. Bridges, M.D. (2002-04-11). "How Does Sickle Cell Cause Disease?". Татаж авсан: 2007-07-23.
- ↑ D.L. Imes, L.A. Geary, R.A. Grahn, L.A. Lyons (2006). "Albinism in the domestic cat (Felis catus) is associated with a tyrosinase (TYR) mutation" (Short Communication). Animal Genetics. 37 (2): 175. Татаж авсан: 2006-05-29.
{{cite journal}}
: CS1 maint: multiple names: authors list (link) - ↑ MedlinePlus: Phenylketonuria
- ↑ Brivanlou AH, Darnell JE Jr (2002). "Signal transduction and the control of gene expression". Science. 295 (5556): 813–8. PMID 11823631.
- ↑ Alberts B, Johnson A, Lewis J, Raff M, Roberts K, and Walter P (2002). Molecular Biology of the Cell (4th edition ed.).
{{cite book}}
:|edition=
has extra text (help)CS1 maint: multiple names: authors list (link) Control of Gene Expression - The Tryptophan Repressor Is a Simple Switch That Turns Genes On and Off in Bacteria - ↑ Jaenisch R, Bird A. "Epigenetic regulation of gene expression: how the genome integrates intrinsic and environmental signals". Nature Genetics. 33 (3s): 245–254.
- ↑ V.L. Chandler (2007). "Paramutation: From Maize to Mice". Cell. 128: 641–645.
- ↑ A.J.F. Griffiths, J.H. Miller, D.T. Suzuki, R.C. Lewontin, and W.M. Gelbart (2000). An Introduction to Genetic Analysis. W.H. Freeman and Company.
{{cite book}}
: CS1 maint: multiple names: authors list (link)Chapter 16 (Mechanisms of Gene Mutation): Spontaneous mutations - ↑ Kunkel TA (2004). "DNA Replication Fidelity". Journal of Biological Chemistry. 279 (17): 16895–16898.
- ↑ A.J.F. Griffiths, J.H. Miller, D.T. Suzuki, R.C. Lewontin, and W.M. Gelbart (2000). An Introduction to Genetic Analysis. W.H. Freeman and Company.
{{cite book}}
: CS1 maint: multiple names: authors list (link)Chapter 16 (Mechanisms of Gene Mutation): Induced mutations - ↑ A.J.F. Griffiths, J.H. Miller, D.T. Suzuki, R.C. Lewontin, and W.M. Gelbart (2000). An Introduction to Genetic Analysis. W.H. Freeman and Company.
{{cite book}}
: CS1 maint: multiple names: authors list (link)Chapter 17 (Chromosome Mutation I: Changes in Chromosome Structure): Introduction - ↑ The Use of Model Organisms in Instruction - University of Wisconsin: Wisconsin Outreach Research Modules
- ↑ NCBI: Genes and Disease
- ↑ T. Strachan and A.P. Read (1999). Human Molecular Genetics 2 (second edition ed.). John Wiley & Sons Inc.
{{cite book}}
:|edition=
has extra text (help)Chapter 18: Cancer Genetics - ↑ H. Lodish, A. Berk, L.S. Zipursky, P. Matsudaira, D. Baltimore, and J. Darnell (2000). Molecular Cell Biology (4th edition ed.).
{{cite book}}
:|edition=
has extra text (help)CS1 maint: multiple names: authors list (link) Chapter 7 (Recombinant DNA and Genomics): 7.1. DNA Cloning with Plasmid Vectors - ↑ H. Lodish, A. Berk, L.S. Zipursky, P. Matsudaira, D. Baltimore, and J. Darnell (2000). Molecular Cell Biology (4th edition ed.).
{{cite book}}
:|edition=
has extra text (help)CS1 maint: multiple names: authors list (link) Chapter 7 (Recombinant DNA and Genomics): 7.7. Polymerase Chain Reaction: An Alternative to Cloning - ↑ T.A. Brown (2002). Genomes 2 (2nd edition ed.).
{{cite book}}
:|edition=
has extra text (help)Section 2 (Studying Genomes), Chapter 6 (Sequencing Genomes): 6.1. The Methodology for DNA Sequencing - ↑ T.A. Brown (2002). Genomes 2 (2nd edition ed.).
{{cite book}}
:|edition=
has extra text (help)Section 2 (Studying Genomes), Chapter 6 (Sequencing Genomes): 6.2. Assembly of a Contiguous DNA Sequence - ↑ Post-Human Genome Project Progress & Resources
See also
[засварлах | кодоор засварлах]Journals
[засварлах | кодоор засварлах]- American Journal of Human Genetics
- American Journal of Medical Genetics
- Annals of Human Genetics
- European Journal of Human Genetics
- Genome Research
- Genomics
- Genetics
- Heredity
- Human Molecular Genetics
- Journal of Genetics
- Journal of Human Genetics
- Journal of Medical Genetics
- Nature Reviews Genetics
- PLoS Genetics
External links
[засварлах | кодоор засварлах]![]() |
Wikibooks: Genetics – Сурах бичиг, зааврууд |

- Bilguun.alt/Ноорог/Генетик Open Directory Project төслийн вэбсайт дахь